Offshore Wind Farm

Background
In 2022, British wind farms reported a record contribution to national electricity, at 26.8% ​[1]​, with offshore wind being responsible for 14.1% respectively, thus, highlighting the significance of these technologies to the existing and future energy network. In a global context, wind power capacity grew by 94
Production of energy using offshore wind has steadily increased since the inception of the technology, with Offshore Wind energy capacity increasing over 2500% between 2009 and 2021 [2].

Figure 1: - Offshore Wind Capacity from 2009-21
Furthermore, the technologies are also improving in capacity and efficiency, with typical turbines in 1985 being rated around 0.05MW capacity compared to newer projects which can achieve 3-4MW and 8-12MW rated power [3] for onshore and offshore projects, respectively. Hornsea 2 in the North Sea off the coast of Yorkshire is the largest offshore wind project, with 165 wind turbines outputting a rated power of 1.3GW, which is enough to power 1.4 million UK homes [4].
Due to the rapid expansion of the offshore wind sector, especially in Scotland, the economic benefit of investment into the offshore wind sector is becoming more lucrative, with private investment expected to see £155bn of private investment between 2022 and 2030 along with the provision of over 65,000 new jobs [5].
Operating Principles
1. Rated Power
Energy production from wind power is the extraction of kinetic energy from the moving air. The kinetic energy of any mass travelling at a given velocity is given by:
Where:
m = Mass (kg)
v = Velocity (m/s)
E = Energy (J)
Mass, m, is also equal to:
​
Where:
m = Mass(kg)
ρ = Density (g/cm3)
V = Volume (m3)
Therefore, the kinetic energy of the wind can be described as:
As Power is energy per unit time, considering a small time interval, , the volume passing through the swept area of the blades can be described as:
Where:
A = Area (m2)
V = Volume (m3)
t = Time (s)
v = Velocity (m/s)
This allows the extractable power from the wind to be described as:
Adding in the coefficient of power,, which is the ratio of how efficiently the wind turbine converts wind energy to electricity, the relationship for the power that can be produced by a given turbine (often referred to as the Rated Power) is given by:
​
​
2. Betz Limit
From this principle, German physicist Albert Betz calculated a maximum efficiency for a wind turbine in 1919 [6] to be 59.3%. This theoretical efficiency is based on the principle of Bernoulli’s conservation of momentum within a fluid flow, where:
As the fluid flow, defined by the wind speed and the swept area of the rotor, strikes the blades, the rotation begins, the flow is decelerated, and the wind’s kinetic energy is reduced. A portion of this change in kinetic energy is then converted into electricity.
For 100% efficiency to be achieved, the velocity of the flow would have to be reduced to zero upon exit, which consequently would not allow any more air to pass through the turbine. Furthermore, all the kinetic energy from the wind must be converted to electricity. This is not achievable in practice as the turbine.
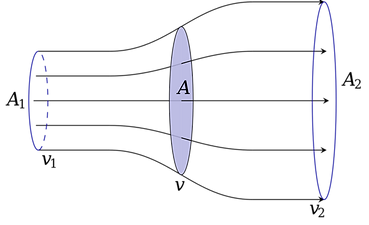
Figure 2: Conservation of Momentum in Fluid Flow
It would require an ideal rotor with infinite blades with no drag. Typically, wind turbines today, such as the Siemens SWT-3.6-120 can achieve a of ~ 43% [7]. However, these values can range from 35-45% [7] dependent on conditions.
Turbine Design
​A wind turbine’s design varies depending on the location and desired power output.
Blade Configurations
The most common design in offshore wind farms is a 3-blade horizontal axis wind turbine (HAWT). The 3-blade design can achieve 80% of the Betz limit and benefits from a natural balance from the 3-bladed rotor, resulting in less vibration [6].
Other blade configurations, such as 2-blade or 1-blade turbines, offer advantages such as higher efficiencies and less requirement for the material. Still, they are limited in their power outputs and suffer from a higher degradation rate due to greater vibrations during rotation [6].
These designs can be compared using a metric called the Tip Speed Ratio or TSR, which is the ratio of the rotation speed of the blades' tip relative to the local wind speed. The TSR is calculated using:
Where:
= Rotational Velocity (rad/s)
R = Rotor Radius (m)
V = Wind Speed (m/s)
Upon comparing the TSR for different blade configurations against the power coefficient of other designs, the 3-bladed rotor yields the greatest power coefficient at around 55%.

Figure 3 - 3-blade HAWT [12]
Types of Installation
Offshore wind turbines are installed in either a fixed position, called fixed offshore wind or attached to a floating platform, called floating offshore wind.
1. Fixed Offshore Wind
In a fixed offshore wind farm, the turbine tower is drilled into the seabed, fixing the turbine. The tower's design may vary between monopile, suction monopile, tripod, jacket, and gravity-based towers depending on the depth of insertion into the water and the turbine size. Fixed offshore wind features are a more familiar technology, with the UK aiming to have 50GW of fixed offshore wind capacity by 2050 and only 5GW of floating power [10]. Figure 6 illustrates the different choices for turbine towers for a fixed offshore wind installation.
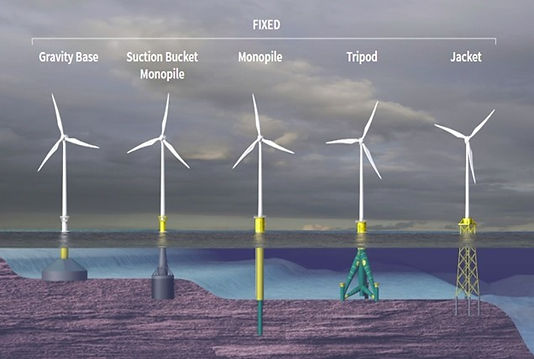
Figure 4 - Fixed Offshore Wind

Figure 5 - Power Coefficient vs TSR
2. Floating Offshore Wind
Floating offshore wind operates similarly to fixed offshore wind, except the turbine tower is not drilled directly into the seabed. The main benefit of floating offshore wind is that it can be placed further offshore, with greater wind speeds. This type of turbine technology uses a Floating Offshore Wind Platform (FOWP), a concrete or steel structure anchored to the seabed by flexible anchors or steel cables [7]. The platform design depends on the sea, seabed conditions, wind speeds and turbine size. Other factors which can influence the platform selection are manufacturing and harbour facilities [7]. The four main platform types that are used in the floating offshore wind industry are the Barge, Semi-submersible, Spar and the Tensioned Legs Platform (TLP) [7]
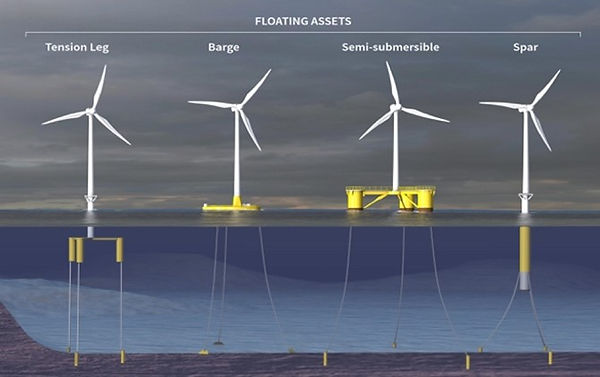
Figure 6 - Floating Offshore Wind
Turbine Spacing
Turbine spacing is important in extracting the greatest kinetic energy from the wind. It is also an important economic factor, as the further the turbines are spread apart, the greater the fuelling costs for operations and maintenance ships. Turbines are laid out in grid-like arrays which consist of several rows and columns. The number of rows and columns depends on the wind farm's desired capacity and the land available for development. There are many different methods of optimization for the layout of the array to achieve.

Figure 7: Wind Turbine Spacing [17]
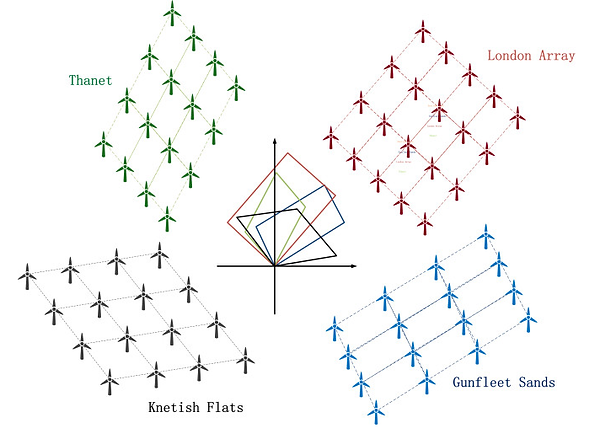
Figure 8: Wind Turbine Spacing [18]
The most common approach is to use computer modelling software to optimise the spacing between each turbine for the given area. Recent research into the Optimal Turbine Spacing in Fully Developed Wind Farms [8] suggests that the optimal average turbine spacing is around 15D in the streamwise direction where D is equal to the diameter of the rotor. This finding disagrees with other relevant literature, which suggests spacing of 6D in the streamwise direction and 3D in the spanwise direction [9]. Overall, the literature within the field of turbine spacing suggests discrepancies between the most optimal layout for an Offshore Wind Farm.
References
[1] National Grid, "Britain produced record amount of wind power in 2022," Reuters, 2023.
[2] L. Fernandez, "Global capacity of offshore wind power 2009-2021," Statista, 2023.
[3] IRENA, "Wind Energy," IRENA, 2022.
​
[4] Orsted, "Hornsea 2, the world’s largest windfarm, enters full operation," Orsted, 2022.
​
[5] R. Norris, "Offshore Wind Industry Council media release," renewableUK, 2022.
​
[6] D. R. M. Andres, "Betz Limit," Saint Louis University, 1st September 2010. [Online]. Available: reuk.co.uk.
​
[7] Siemens Gamesa, "Siemens SWT-3.6-120 Onshore," Wind Turbine Models.
[8] Educational Innovators, "Understanding Coefficient of Power and Betz Limit," [Online]. Available: http://cdn.teachersource.com/downloads/lesson_pdf/betz_limit_0.pdf.
[9] K. Adeyeye, N. Ijumba and J. Colton, "The Effect of the Number of Blades on the Efficiency of A Wind Turbine," in 2021 11th International Conference on Future Environment and Energy, 2021.
[10] Great Britain and Northern Ireland, "Offshore wind," Department for International Trade, 2022.
[11] Iberdrola, "Floating offshore wind power: a milestone to boost renewables through innovation," Iberdrola.
[12] J. Meyers, "Optimal turbine spacing in fully developed wind farm boundary layers," Wind Energy, 2012.
[13] N. Ali, N. Hamilton, R. B. Cal and D. DeLucia, "Assessing spacing impact on coherent fearures in a wind turbine array boundary layer," Wind Energy Science, vol. 3, no. 1, pp. 43-56, 2018.
[14] Wind Europe, "France commits to 40 GW offshore wind by 2050," Wind Europe, 31 March 2022. [Online]. Available: https://windeurope.org/newsroom/news/france-commits-to-40-gw-offshore-wind-by-2050/.
​
[15] C. Markfort and P. Shivendra, "Experimental investigation of aerodynamic characterisitics of bat carcasses after collision with a wind turbine," Wind Energy Science, vol. 5, no. 2, pp. 745-758, 2020.
[16] Acteon, "FLOATING WIND: WHAT ARE THE MOORING OPTIONS? - A Q&A WITH KENT LONGRIDGE," Acteon.
​
[17] R. McKenna, S. Pfenninger, H. U. Heinrichs and J. Schmidt, "High-resolution large-scale onshore wind energy assessments: A review of potential definitions, methodologies and future research needs," Renewable Energy, vol. 10, no. 182, 2021.
[18] Z. Yong and Q. Shengli, "A Practical Optimization for Offshore Wind Farm Layout," Renowind Energy Technology, Beijing .